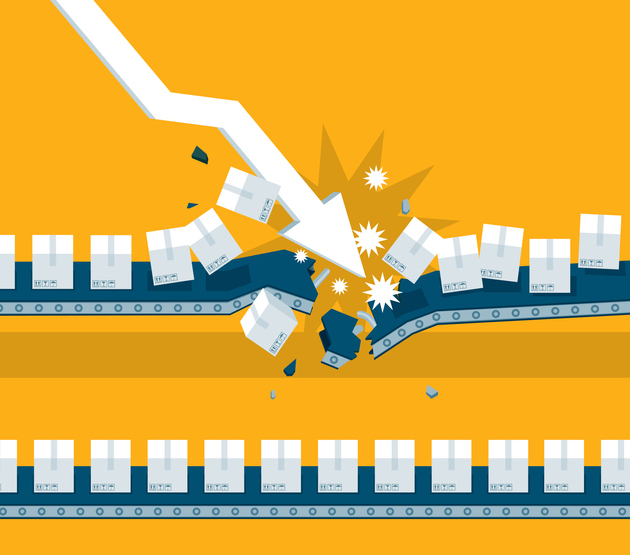
In laboratories around the globe, scientists are diligently researching the limitless potential of advanced therapeutic medicinal products (ATMPs). These groundbreaking therapies are changing the face of healthcare by harnessing the potential of the human body for healing. Using cells, proteins, antibodies, tissues and nucleic acids as “living” therapies is simultaneously promising, but also brings unique challenges from a manufacturing, supply chain, and patient treatment standpoint. The advent of ATMPs is a foundational milestone, the growth of which is comparable to the rise of the microprocessor in terms of its transformative potential for effecting change on humanity. The “holy grail” of ATMP-based treatments is to provide cures for some of the world’s most debilitating disease states, but for this to occur, a unified front is needed to democratize these therapies – collaboration from industry, academia, supply chain and, indeed, clinicians and hospitals is paramount to ensuring safe manufacturing and transit.
The reasons for ATMPs garnering huge attention from the scientific community are well justified — ATMPs are now starting to demonstrate curative potential, not just symptomatic treatment, for a litany of rare or intractable diseases. These therapies also come in various forms — some can be classified as cell therapies, others as gene therapies, gene-modified cell therapies, nucleic acid drugs and other therapies including personalized cancer vaccines (PCVs), tissue engineered products, CRISPR-edited therapies and even radioligand therapies, among others. Still, despite their immense promise, additional research is needed before we fully understand and take advantage of the curative potential conferred by ATMPs.
ATMPs have a long history but were not considered treatments in their earliest forms. In the 1950s, the concept of a “cell therapy” did not exist. Rather, experiments were being conducted to treat radiation victims with bone marrow transplants, but failed due to an unknown reason that we now know to be an immune response-mediated rejection. In 1956, the first ever bone marrow transplant was performed, with surprising results – an identical twin was the key to a successful engraftment, which led soon to the discovery of human leukocyte antigens (HLA), and the understanding that therapies from biological sources needed to match the donor and recipient HLA-type. Around the same time, Canadian researchers Till and McCulloch were performing similar experiments in irradiated mice and found injected bone marrow produces small nodules in the spleen. Since then, an immense body of work has shown that these cells can in fact self-renew and differentiate into multiple lineages, which led to the term “stem” cells, alluding to their being the origin of all tissues in our body.
Today, we have reached new heights in our understanding of the complex signaling pathways and mechanisms of action that contribute to human disease. There are many milestones to speak of in the ATMP space, but most notable among them is a staggering 34,400 chimeric antigen receptor (CAR)-T cell therapies have been delivered to patients as of October 2023. There are now 34 FDA-approved cell or gene therapies in the commercial pipeline, with hundreds more in development. Today, there are six commercially approved CAR-T therapies on the market, and in some countries and regions, they are reimbursed by government health authorities, enabling their use in larger patient populations. Thanks to key pioneers in the field of ATMPs, we can now confidently report that therapies like CAR-T cells are forming a new foundation for cancer treatment.
Of course, the story does not end here. Numerous other ATMPs are now being developed with greater potential for human treatment, such as allogeneic therapies, which can be delivered to an even larger patient population. These require larger bioreactors and healthy donor cell material but offer the promise of lower cost and wider accessibility. Similarly, a large body of work is being done to create therapies for the treatment of rare or orphan diseases. These include gene therapies based on adeno-associated viruses (AAV) that are being developed for both prevalent and rare disease states, such as congenital blindness, sickle cell disease, hemophilia B, dystrophic epidermis bullosa, and even diabetes and spinal muscular atrophy. As examples of commercial successes in this area, Luxturna, Zolgensma, Lyfgenia, and Vyjuvek are approved gene therapies for some of these rarest indications. More recently, new non-viral methods of production are being explored to avoid speculation around the possibility of replication competency that could arise from the use of viral vectors during production.
For example, Vertex Pharmaceuticals and CRISPR Therapeutics made history by gaining the first FDA approval for a CRISPR-based drug, exagamglogene autotemcel (Casgevy) for the treatment of sickle cell disease with vaso-occlusive crisis, coinciding with a record number of 14 review designations awarded by the FDA to CRISPR-based therapies in 2023, according to data and analytics firm GlobalData. In 2023, a record six orphan drug designations, four fast track designations, two regenerative medicine advance therapy designations, and a single rare pediatric disease designation were granted, and priority review was awarded to 10 different CRISPR drugs, representing a 55% increase from 2021 and 2022. These numbers indicate an increased priority and appetite for ATMPs by regulators and manufacturers.
At the end of 2023, there were more than 100 different approved gene, cell, and RNA therapies throughout the world, with over 3,700 more in development. Nucleic acid therapies gained infamy during the pandemic as a rapid and effective way to resolve a key global crisis through mRNA technology, but they also exposed weaknesses and revealed opportunities for new ATMP modalities. In more recent times, mRNA therapies are being used to create personalized cancer vaccines (PCVs), demonstrating their continued usefulness to larger patient populations with severe illnesses in a post-Covid world. Similarly, the advent of nuclear medicines, which has existed for decades, has brought a new contender to the sphere of radioligand therapy (RLT). This therapeutic modality is demonstrating efficacy for some of the most intractable solid tumor cancers, such as metastatic castration-resistant prostate cancer.
While the focus for ATMPs has been on treating cancers like B cell lymphomas and leukemias, as well as rare disease like hemophilia B and sickle cell disease, novel application areas are emerging, such as treatments for diabetes, Alzheimer’s, and even cardiovascular disease.
With this excitement comes the realization there is still work to be done to understand the underlying biology behind these ATMPs. As an example, it was recently reported that a small proportion of patients having received CAR-T therapy had also developed secondary cancers in the form of T cell malignancies, which now requires a box label warning. There are also known side effects for CAR-T therapies, which include cytokine release syndrome (CRS), immune effector-cell associated neurotoxicity syndrome (ICANS). Some patients, despite the high levels of response to the therapies broadly seen, do not survive their cancers in the end. Even with these setbacks, it is generally agreed the overall benefits of these ATMPs greatly outweigh their risks. Looking ahead, we must respond to the ever-increasing demand for ATMPs to ensure a consistent supply chain for the patients who require them.
Photo: sorbetto, Getty Images